Projects
Humanitarian Project in Cooperation with AUVA
In recent years, interdisciplinary approaches and innovative technologies have collectively enhanced physical capabilities and brought about profound changes in the daily lives of individuals with amputations. Unfortunately, the advances in limb prosthetics have not benefited individuals in developing and transitional countries to the same extent as in industrialized nations. A similar situation exists in conflict zones. The reasons for this are often significant infrastructural challenges and a shortage of qualified personnel. This presents a dilemma, especially in conflict zones where the number of people with amputations is on the rise, leading to a greater demand for efficient and, above all, cost-effective prosthetics.
Students studying the construction and functionality of myoprostheses provided by AUVA
Pictorial Source: University of Applied Sciences Upper Austria
The Linz branch of AUVA acts as a mediator of second-hand prosthetics in this project. The goal of this project is to support students in developing countries and those who, due to circumstances, had to leave their home country and are now residing in Austria. The education of these students can take place in close collaboration with the universities in their home locations. This approach enables young individuals to gain expertise in the field of prosthetic care, addressing both the infrastructural challenges and the shortage of qualified personnel.
Student conducting the functional test of a myo-prosthesis – Winter School in Medical Engineering
Pictorial Source: University of Applied Sciences Upper Austria
The Department of Medical Engineering and the International Office of the University of Applied Sciences Upper Austria welcome international students to the Winter School in Medical Engineering every year. Participants go through a program that includes lectures, laboratory work, and cultural activities. One focus area of Linz’s medical engineering concerns prosthetics.
QAM – Quadrature Amplitude Modulation
QAM stands for Quadrature Amplitude Modulation. QAM combines both amplitude modulation (AM) and phase modulation (PM) to encode and transmit digital information. By varying the amplitude and phase of the carrier signal, multiple discrete amplitude levels and phase shifts can be used to represent different digital symbols or bits. Quadrature Amplitude Modulation (QAM) is also used in medical imaging devices. In medical imaging, such as Magnetic Resonance Imaging (MRI) and ultrasound, complex modulation techniques like QAM are frequently employed to encode and transmit digital information within the generated signals. In the field of MRI, for example, QAM can be used in image reconstruction and processing. The received signals from the magnetic resonance scanner are modulated and demodulated to extract information about tissue properties and generate high-quality images. Similarly, QAM is utilized in ultrasound imaging to modulate and demodulate the high-frequency ultrasound signals. This enables precise visualization of tissue structures and the capture of diagnostically relevant information. In both cases, QAM serves to enhance the efficiency and quality of data transmission and image formation in medical imaging devices. The setup in the image displays laboratory equipment used to implement a QAM modulator.
The objective of this project is to establish a Science-Lab platform to develop and test various applications. An example of this is a band-pass filter, comprised of a QAM modulator, a QAM demodulator, and a complex valued low-pass filter as shown below.
TENS -Transcutaneous Electrical Nerve Stimulation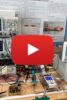
TENS (Transcutaneous Electrical Nerve Stimulation) is a therapeutic method in which electrical impulses are used through the skin to alleviate pain. Special TENS devices are used to generate low-intensity electrical currents applied to the skin through electrodes. The electrical impulses stimulate the nerve fibers in the skin and underlying tissues, which can result in muscle contractions. TENS is commonly used for the treatment of chronic pain conditions such as muscle and joint pain, back pain, arthritis, neuralgia, and postoperative pain. TENS can also be used to train and strengthen weakened muscles. The applications of TENS are diverse. The TENS Science Lab platform enables students to develop their own applications. It involves an existing TENS device that has been technically prepared to integrate external hardware components. Isolation protection circuits prevent the existing TENS device from being damaged during experimentation. Additionally, it provides control and monitoring signals that can be displayed for analysis purposes.In biomedical engineering studies, TENS (Transcutaneous Electrical Nerve Stimulation) plays a significant role. In addition to theoretical understanding, students are taught the practical application of TENS, as well as the approach to research and development (see TENS Science Lab experimental platform above)
EMG/ECG/EEG – Amplifier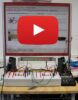
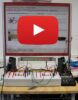
EMG (Electromyography) is the recording of the electrical activity of skeletal muscles. It measures the electrical potentials generated during muscle contraction to obtain information about muscle activity, strength, and function. EMG is commonly used in rehabilitation, sports medicine, and motion analysis. EKG (Electrocardiography) is the recording of the electrical activity of the heart. It measures the electrical potentials generated during the cardiac cycle to obtain information about heart rhythm, heart rate, conduction system, and possible heart problems. EKG is used for the diagnosis and monitoring of heart diseases. EEG (Electroencephalography) is the recording of the electrical activity of the brain. It measures the electrical potentials generated by neurons in the brain to obtain information about brain activity, brain wave patterns, epilepsy, sleep disorders, and other neurological conditions. EEG is used in neurology, sleep research, and brain research.The amplifiers of these electrical signals must meet certain requirements regarding noise suppression and inherent noise. External disturbances and interferences need to be effectively suppressed. This can be achieved through a combination of measures such as a differential input configuration, common-mode rejection (CMRR), shielding techniques, and filtering. The higher the CMRR value, the better the amplifier’s ability to suppress unwanted interference signals. The differential amplifier should have low inherent noise to improve the signal-to-noise ratio (SNR). Low inherent noise enables accurate detection of weak signals and reduces the likelihood of artifacts and disturbances. The bandwidth of the differential amplifier must be sufficient to capture the relevant frequencies of the EMG, ECG, and EEG signals. This is particularly important when the signal exhibits rapid changes or covers a wide frequency range. Adequate bandwidth ensures precise and accurate signal capture with minimal distortion. Additionally, the differential amplifier needs to have high input impedance to prevent signal distortion due to loading.
With the EMG/ECG/EEG Science Lab platform, students can develop and test their own signal amplifiers. Furthermore, all signals can be visualized and made audible to deepen the understanding of the nature of the signals (see EMG/ECG/EEG Science Lab experimental platform above)
Prostheses – Gripping pattern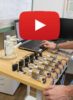
In prosthetic hands, there are various gripping patterns that help users to grasp and manipulate objects. Cylindrical grip: The thumb is opposite to the other fingers and wraps around the object like a cylinder. This grip is suitable for objects such as glasses or bottles. Key grip: The thumb rests on one side of the object while the remaining fingers lie on the opposite side. This grip is useful for holding flat objects like keys or cards. Three-point grip: The thumb and two other fingers form a grip around the object. This grip provides good stability and is suitable for holding heavy or bulky objects. Precision grip: The thumb and fingertips are used to grasp small objects with great precision. This grip is suitable for tasks that require fine motor skills, such as gripping a pen or buttoning clothing. Lateral grip: The side edge of the hand and the fingers are used to grasp objects. This grip is suitable for wider objects such as books or tablets. These gripping patterns serve as basic examples, and depending on the type and functionality of the prosthetic hand, additional specialized gripping patterns may be available to provide users with diverse grasping options.
With the Prostheses Science Lab platform, gripping patterns are simulated using a 3D prosthetic hand (left) driven by five brush-less dc-motors (right). Students investigate their utility in the daily lives of prosthetic wearers (see Prostheses Science Lab experimental platform above)
Socket – Liner
A liner is a component of a prosthetic device that is placed between the amputee’s residual limb and the prosthesis. It is made of soft, flexible silicone material and serves to provide a comfortable and secure fit. The silicone liner is pulled over the residual limb and forms a layer between the limb and the hard surface of the prosthesis. It creates a soft, cushioned surface that protects the limb from pressure points, friction, and skin irritation. Additionally, the silicone liner can help improve the grip of the prosthesis by providing better adhesion and stability. Liners are available in various shapes and sizes to accommodate the individual needs of the amputee. They can be custom-made or prefabricated and are typically replaced regularly to ensure optimal fit and functionality. In the present project, various materials are being investigated for their suitability as liners. The main focus is on breathability, material softness, and compression under load. In order to meet all requirements, prototypes of multilayer liners are being created and tested. Spacer fabrics play an important role in this process.The image above shows a patient with a below-knee amputation. A neuroma has formed in the area of the severed peroneal nerve. This area is perceived as a painful point. Suitable liners are particularly important in this case as they can help alleviate the patient’s pain while wearing the prosthesis.
Energy Harvesting – Prostheses
Energy harvesting plays a significant role in leg prosthetics. It refers to the process of capturing and utilizing energy from the surrounding environment to power various components of the prosthetic limb. In the context of leg prosthetics, energy harvesting is particularly important for improving the functionality and usability of the prosthetic limb. By harnessing energy from sources such as the motion of the user’s steps or the mechanical forces exerted during walking or running, energy harvesting systems can generate electrical power to drive components like motors, sensors, or electronic systems within the prosthesis. This can enhance the performance of the prosthesis, enable additional features, and potentially extend the battery life or eliminate the need for external power sources. Energy harvesting technology has the potential to enhance the autonomy and convenience of leg prostheses by reducing the reliance on external power and improving the overall user experience. It can contribute to increased mobility, reduced maintenance requirements, and greater freedom of movement for individuals using leg prostheses.The image above shows a shoe sole equipped with piezoelectric elements. With each rolling movement of the sole on the ground, the piezoelectric elements deform and generate a small amount of electrical energy. The greater the number of elements in the sole, the greater the “collected” energy.
Case study: leg prosthesis that sends feelings to its wearer 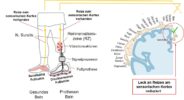
The patient Wolfgang R. from Upper Austria underwent “Targeted Sensory Reinnervation” by OÄ Dr. Eva-Maria Baur and OA Dr. Thomas Bauer at the University Clinic for Reconstructive Plastic and Aesthetic Surgery in Innsbruck which is a cooperation partner of the FH Upper Austria. During this procedure sensorial nerve endings of the original foot were reactivated to serve as an indicator for pressure on the sole of the prosthetic. The main goal of the operation was to decrease pain from neural scarring (neuroma) by redirecting the nerves.Due to the neural interface the patient has feeling on the sole of the prosthetic foot and therefore manages much better to recognize the nature of the ground he is walking on. There is also more awareness of obstacles, which greatly reduces the risk of falling. The re-established transfer of information also contributes to a more natural integration of the prosthesis into the body concept of the patient and, in this case, has led to the complete disappearance of previous long lasting pains. Information for media see DOWNLOAD
Phantom pain investigations due to meteoropathy
Many amputees may suffer from limb phantom pain symptoms ranging from mild to extreme pain felt in the area of the amputated limb. Residual nerve endings (neuroma) continue to send action potentials to the brain. This leads to a kind of feeling as if the limb were still there. Often, the feeling is so strong or even unbearable that it is being felt as limb phantom pain. Affected people also complain about the limb phantom pain strongly correlated with weather deterioration – a phenomenon which is still unexplored. The goal of the objective project is to find out the primary causes responsible for meteoropathy.
Surface EMG pattern recognition for reliable real-time control of myo-prostheses
As the classification of limb gestures and movements is partly possible with pattern recognition methods, prostheses are being developed using this strategy. However experience has taught us that changes of skin-electrode properties such as skin artefacts due to sweating, skin movement or electrode displacement increase the error rate significantly. Unfortunately, this makes a prosthesis using pattern recognition methods unreliable so that users often wish more simple and robust control methods instead of sophisticated ones. The goal of this project is to design and test surface electrodes delivering signals broadly independent from skin artefacts.
Pattern recognition to improve recognition of co-contraction
Well established myo-prostheses use co-contraction commands ideally given by s1(t) = s2(t) to route the EMG-control-signal to the different joint’s motors. Although the method is rather robust once it is learned some users may still have problems to evoke predefined command signals. As the characteristics of real signals depending on the amount, orientation and scarring of the residual muscles strongly vary mainly elderly people can have difficulties to perform the command signals s1(t) = s2(t). The goal of this project is to improve the individual’s reliability of co-contraction by using pattern recognition methods.